News Story
Unlocking the Mysteries of Fire ... in Space
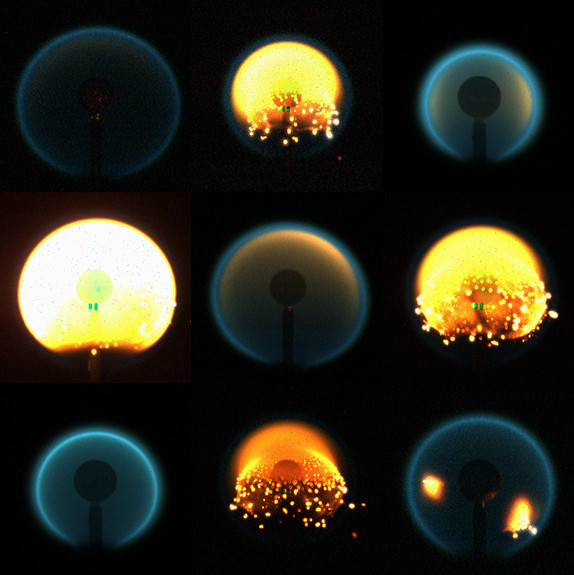
A composite of flame images from nine different tests of the flame design experiment. Photo: Space Flames/NASA
Fire.
Humans have used it as a tool for uncounted generations. Once some curious and brave soul puzzled out how to grab a bit of burning grass from a lightning strike, the rest has been history.
For most of that time, we have employed fire in its most basic form: as an open flame, most often for cooking, for heat, for ritual. Eventually, we learned enough about fire to harness it into chambers, using it to produce the power that has enabled the breakneck pace of modern industry and global transportation.
Though a full understanding of fire behavior eludes us still, combustion, in its many forms, remains the smoldering heart of daily life.
It is natural, then, that as humans have moved into space, we have taken fire with us—and it may well be in space where humans finally unlock the elusive secrets of how fire behaves.
On Earth, fire is complicated. Burning fuels create heat, which creates convection, hauling air and other unevenly sized molecules haphazardly upward. Ever-present is the influence of gravity, drawing heavier gases down. This push-and-pull causes flames and their vapors to eddy unpredictably in all directions.
“When you look at a candle flame and it flickers, it’ll do that even if there’s no draft,” explains Dennis Stocker, project scientist with the Advanced Combustion via Microgravity Experiments (ACME) program at the NASA Glenn Research Center in Cleveland, Ohio. “And it does that because of gravity. In microgravity, flames don’t flicker.”
In the study of flame behavior and design, the average combustion researcher likely does not have a role on an experiment aboard the International Space Station (ISS).
Peter Sunderland has three.
New Insights into Ancient Technology
A professor of fire protection engineering at the University of Maryland’s A. James Clark School of Engineering, Sunderland has been working in microgravity research for decades. He arrived at NASA Glenn in 1996 after working with Ingersoll-Rand and United Technologies; he has been at UMD since 2004, when James Quintiere, professor emeritus of fire protection engineering, heard Sunderland present at a conference and insisted he apply for an opening at Maryland’s flagship university.
Sunderland’s current research involves leading one and co-investigating two other experiments aboard ISS. While they individually cover a wide range of questions and potential future applications, the projects all have one essential purpose: to build a more complete picture of how fire and flames behave—from an engineer’s perspective.
“Part of what we’re learning is how much we didn’t know,” Sunderland says.
“The big picture is to improve our understanding of combustion,” says Stocker, who worked with Sunderland at NASA Glenn and continues to collaborate with Sunderland on his ISS experiments. “If you can model it, that computationally suggests that yes, we do understand what’s happening.”
Sunderland is involved in three experiments: a burning rate emulator, how to design flames to reduce or eliminate the formation of soot, and eerie-blue “cool flames” that could one day improve the design of combustion engines. Sunderland is the principal investigator on the cool flames project, and co-investigator on the others.
“One of the most interesting things to me is how long fire has been around, yet we’re still discovering so many new things about it,” says Kendyl Waddell, a Clark Doctoral Fellow and second-year Ph.D. student in Sunderland’s lab. “Basic concepts we have about fire may not necessarily be true in microgravity, and it’s interesting to see the differences and try to figure out why it’s different.”
By way of example, Waddell says a current focus is why flames with differing oxygen and fuel concentrations and flow rates still extinguish at the same temperature in space—it was expected that the flames would extinguish at different temperatures. “We’re still trying to work out why that is,” Waddell says.
Among the combustion research community, Sunderland’s work often receives a lot of interest; fire-in-space could have negative connotations, but Sunderland says other academics enjoy learning about it because it’s so unusual.
“Peter is one-of-a-kind,” offers Richard Axelbaum, a frequent collaborator and the Stifel & Quinette Jens Professor of Environmental Engineering Science at Washington University in St. Louis. “He’s so creative at applying the knowledge he’s acquired over the years and using it to inform experiments to study flames in microgravity.”
A Safer Space
Fire-related tragedies have afflicted the space program since its earliest days. A 1967 cabin fire during a launch rehearsal of the Apollo 1 mission killed all three of its crew; a 1997 fire aboard the Mir space station created thick smoke and flames that proved unexpectedly difficult to extinguish.
To be clear, all of Sunderland’s experiments, and the rest of the ACME investigations, are conducted under completely controlled conditions aboard ISS in a special enclosure known as the Combustion Integrated Rack (CIR). One experiment in particular aims to make living in space even safer than it is today.
“Astronauts love fire,” Sunderland says—meaning that, in general, they’re all quite worried about it and keenly interested in the outcomes of flammability studies.
Launched in 2017, the burning rate emulator experiment was designed to imitate how different common materials found aboard the space station might ignite and burn in microgravity. Conducted throughout 2019 and 2020 and wrapping up in early 2021, the experiment, which includes Quintiere, burned gaseous ethylene and methane under a variety of conditions to emulate how real-life fuels like wire insulation, Velcro, plastic, oil, cleaning solutions, trash, paper, and fabric may burn.
Though results are still months away pending analysis of two years of data, Sunderland says an early observation is that items presumed to be of low flammability hazard may actually present more of a danger than previously believed.
“We seem to be seeing that a lot of materials where people said, ‘Don’t worry, it won’t burn in space,’ do actually burn,” Sunderland says. “The tests that they’ve been relying on perhaps haven’t been conservative enough. But it’s true that overall, things are less flammable in space than on Earth.”
One aim of the project is to create a flammability index of materials used aboard ISS; astronauts’ shirts, for instance, now made of cotton, may be swapped out for polyester if results show that it is safer in space.
“There are a lot of materials that can be used in space, and hopefully with this work we can help guide those decisions,” Sunderland says.
A Well-Made Fire
As a term, “design” is synonymous with “looks nice” and/or “functions well.” If you look around, it’s probably easy to identify numerous objects and systems that have sprung from thoughtful design: clothing, furnishings, toys and games, apps, automobiles, transportation networks.
Flame generation, on the other hand, doesn’t seem to involve much in the way of design: start a fire, give it some fuel, and away it goes. This is how it works for most combustion processes today: ignition of a fuel occurs in ambient or slightly oxygen-enriched conditions, and the resulting flame generates heat energy to perform work.
Unfortunately, flames burning in these conditions also often generate carbon-rich soot particles, a process that is still poorly understood. Soot is problematic for a variety of reasons; in addition to being a major contributor to air pollution and a hazard to human health, soot severely reduces the efficiency of combustion engines and power plants. Accumulations can damage mechanical parts.
The flame design project aboard ISS is a deeper inquiry into the conditions under which soot forms, and how to “design” a flame to reduce or eliminate soot. With Axelbaum and David Urban, a microgravity combustion science researcher at NASA Glenn, the project kicked off in 2014 with terrestrial experiments, then moved to the ISS in 2017. Experiments will continue through 2021.
The fundamental idea behind flame design: if nitrogen can be removed from air and mixed with the fuel to be burned, then the resulting carbon atoms in the flame will be so diluted as to make the flame soot-free.
Sunderland’s current research involves leading one and co-investigating two other experiments aboard the International Space Station.
While they individually cover a wide range of questions and potential future applications, the projects all have one essential purpose: to build a more complete picture of how fire and flames behave—from an engineer’s perspective.
“We want to make engines that are inherently soot-free, no matter who is stepping on the pedal,” Sunderland says.
Back to the candle, and its characteristic yellow color: the bright hue is due to heat radiating from glowing soot particles, generated from carbon in the candle paraffin that evaporates at the wick. Usually, the soot itself will be consumed in the heat of the flame and burn away cleanly. But sometimes, candle flames will emit a thread of dark smoke; these are soot particles that have not been consumed, and are escaping into the air.
“In a car, when you step on the gas to go really fast, [the engine] may not have the ability to burn up the soot before it goes out the exhaust,” Axelbaum explains. “The same thing happens in a power plant; soot often can’t be burned fast enough before it escapes. So flame design is to allow us to have the flame without the soot in the first place, ensuring complete combustion that’s not guaranteed in a typical flame.”
And the kicker: a flame without soot glows blue, but just as hot as any yellow flame—as demonstrated by the “blue whirl” flames discovered in 2016 at UMD.
Despite the tiny size of soot particles, they contain millions of carbon atoms, and the chemical processes behind their formation are incredibly complex. “If you had to write all the chemical reactions that happen, it’s crazy difficult,” Sunderland says.
Part of what makes a tool or technology useful is the ability to predict how it will function in a given set of circumstances. Computer modeling is often employed to do this in many scientific disciplines; from a computational perspective, modeling the behavior of soot formation under normal gravity is, in a word, a nightmare.
In space, by contrast, a flame has only one spatial coordinate: radius.
“The whole flame is a sphere,” Sunderland says. “In normal flames that you test on the ground, the molecules get hot and cold again. Even in the simplest flame, it’s a very complex flow, with complex thermal and chemical dynamics. In a turbulent flame, you have three coordinates, and every time you add a coordinate, you also add a lot of complexity to a computer model.”
The experiments to date have generated not only some surprising observations and measurements, but also some dazzling video footage. In one ISS video, soot particles can be seen forming and burning brightly for a moment before fading and then re-igniting elsewhere in the spherical flame, like so many stars in a tiny galaxy.
Flame design research may also have two potentially important spinoffs.
Axelbaum, who also directs the Advanced Coal and Energy Research Facility (ACERF) at Washington University, says the microgravity research may ultimately result in better carbon capture and sequestration approaches. Since “designed flames” can remove most or all the nitrogen, it becomes easier to capture the remaining carbon dioxide after the hydrocarbon fuels (coal, natural gas) have been consumed. Additionally, removing nitrogen from the air mix that’s used to fuel power plants reduces harmful emissions, which are major contributors to acid rain and air pollution.
Axelbaum is currently working on the design of a new breed of coal-fired power plants that employ compressed oxygen-rich air. By injecting this air in stages in a sequence of combustion chambers, the temperature can be more controlled (allowing for better combustion), and the increased pressure results in increased heat radiation from the water and carbon dioxide molecules in the chamber.
These benefits may never have come to be if it weren’t for fundamental research on flame design and combustion processes, said Scott Hume, a researcher with the Electric Power Research Institute, and a collaborator of Axelbaum’s.
“Computational fluid dynamics have come a long way, and that’s helping to open the door for a better understanding of combustion reactions. But there’s a lot to learn,” Hume says. “Microgravity combustion is something that’s never been looked at. Without the combustion part of these processes being well-understood, we wouldn’t be able to design larger systems that are more efficient, or realize all of the efficiency benefits.”
Second: a better understanding of forest fires and their impacts. Soot particles generated from large-scale forest fires can become quite large, yet still remain suspended for long periods of time in the atmosphere, and thence be transported on air currents around the globe.
“We can get at the mechanism for how these enormous particles form, what’s causing that to happen,” Axelbaum says. “And from there, you can gain a better understanding of their optical properties and how they affect the atmosphere, and develop models of those processes.”
Cool Flames
There are plenty of stories about strange phenomena occurring in space, but the observation of a new type of flame aboard ISS was a truly bizarre moment, and one that set off a flurry of excitement and research.
Though they’d been theoretically predicted, spherical cool diffusion flames, or simply “cool flames,” were first observed forming around liquid droplets during an experiment in 2012; though they have been re-created on Earth with great difficulty, space is still the best and easiest place to create and study them. They were already known from experiments using “premixed” fuels, where oxygen and fuel are already combined prior to combustion—but on the ISS, the flames formed at the boundary between the fuel and the surrounding air, diffusing outward as it burned.
While these cool flames are diffusion flames like candles, they burn at extremely low temperatures, making them so dim as to be near-invisible or just barely glowing a deep blue.
For example, a natural gas burner at high heat on a conventional stovetop can burn at about 2000 Kelvins (around 3100 degrees Fahrenheit); a typical cool flame hovers around 750 Kelvins (900 degrees Fahrenheit). The potential major application of figuring out how to harness these strange flames is, again, for better vehicles.
“If you could burn a cool flame in your house, it would stink so bad: they’ve got lots of formaldehyde,” Sunderland laughs. “But you do want them in engines. You could take the high efficiency of diesel and the low emissions of gasoline and put them together.”
Just launched to ISS in late 2020, the cool flames experiments will get underway in April 2021. Sunderland is again joined by Axelbaum on the project, as well as University of California San Diego combustion researcher Forman Williams. Until August, astronauts will help researchers test a variety of gas fuels, including ethane, propane, and butane.
“No one has ever tried these fuels before, so we’re going to throw everything at it,” Sunderland says. “There are a lot of unknowns. We have a lot we can try because we’re using gases—but it’s a nerve-wracking experiment.”
The chemistry of cool flames is so different from that of hotter flames that a key aim of the microgravity research will be to understand the particular processes ongoing in these very dim, quiet flames.
To this point the only spherical cool flames have been with droplets, which never achieve a necessary stability. Sunderland is hopeful that the upcoming experiments will generate flames that last minutes, for a chance to make observations and take measurements on steady state flames.
Sunderland and Axelbaum say the immediately obvious application would be in a new type of engine that employs a homogenous-charge compression-ignition (HCCI) strategy. Similar to a diesel engine, HCCI engines compress a low-fuel/high air mix already in the chamber to the ignition point. However, the use of gasoline as a fuel has proven difficult in current prototype designs, since the temperature must be just right.
Sunderland explains that if cool flame chemistry were better understood, existing pockets of these low-temperature flames throughout the HCCI air-fuel mixture could be used as a kind of catalyst to control the timing of higher-temperature combustion.
“We don’t think cool flames would ever be an energy producer,” Sunderland says, “but in an HCCI engine, cool flames could be the fuse that ignites the flame.”
Though he acknowledges there are many links between the results of the foundational science experiments aboard ISS and how the data may actually benefit automotive design, Sunderland says that here again, the ability to create a complete and working computer model of cool flame generation is a key aim.
“It could be the missing link,” he says. “If we get good measurements, then we could get a model that works in a simple system; automakers may be able to take that to study other factors, or incorporate it into more complex models to improve their engines.”
— Story by Michelle Z. Donahue
Published February 10, 2021