News Story
Rubloff and colleagues publish work on improving solid-state battery interface performance
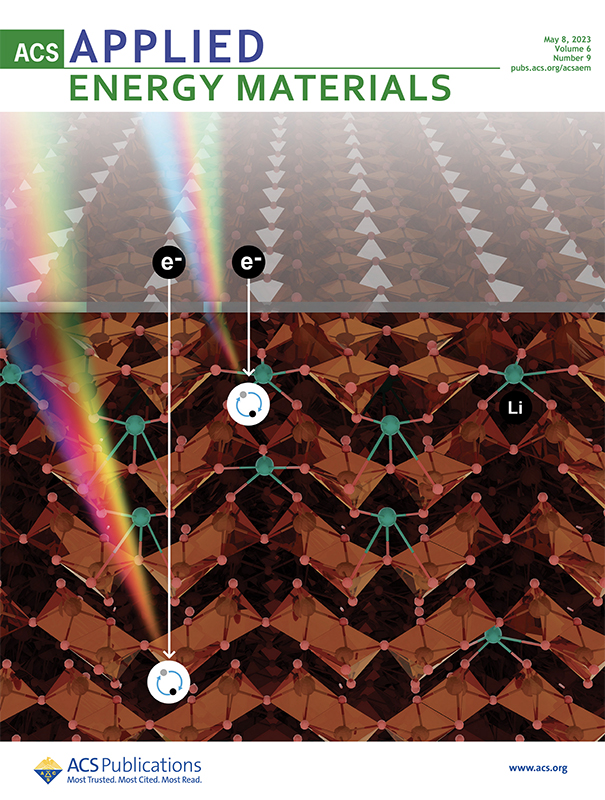
The cover of the journal depicts DRCLS electron cascade, impact ionization, recombination, and light emission generated by an incident electron beam passing through an electrolyte overlayer into a V2O5 crystal with nanoscale depth resolution to distinguish between electronic properties at the interface versus the V2O5 bulk. Image courtesy American Chemical Society.
Research into solid-state batteries is growing in many directions with different chemistries, but fundamental trade-offs between energy and power persist. As battery research evolves toward more advanced schemes and mesoscale designs, using 3D architectures that enhance the surface area for reactions is an obvious way to improve device performance at high power. However, as surface area increases, the role of interfaces in the performance of batteries becomes increasingly critical. New techniques are needed to examine the microscopic interphase formation between cathode and inorganic electrolytes.
Now, an article written by nine researchers at three universities—including three from the University of Maryland—introduces a new combination of techniques to correlate chemical and electronic structure properties of the electro-chemical cathode/electrolyte interface before and after ionic transfer process.
“Lithium Spatial Distribution and Split-Off Electronic Bands at Nanoscale V2O5/LiPON Interfaces,” is the cover article of the May 8, 2023 issue of the American Chemical Society’s Applied Energy Materials journal.
Clark Engineering contributors include Distinguished University Professor Gary Rubloff (MSE/ISR); his Material Science and Engineering Ph.D. student Victoria Castagna Ferrari; and MSE Assistant Research Scientist David Stewart, who works in Rubloff’s group. Authors from the Ohio State University Physics Department include first author Zach Levy, Pablo Rosas, Kalpak Duddella, Micah Haseman and Professor Leonard Brillson (OSU ECE/Physics). Mitchell Walker of the Northwestern University MSE Department also is a co-author.
The challenge
Electrochemical energy storage relies on the exchange of ions across the interface between an electronic insulator (the electrolyte) and a semiconductor (the electrode). The rate at which this transfer occurs depends on several factors (such as the reaction energy). Most important is the presence of charged defects near this interface—namely, Li ions in the electrolyte, neutral/charged Li interstitials in the electrodes, free electrons, and corresponding vacancies.
In a traditional solid-state electronic device, this interface is well described by the band theory of electrons and holes. For solid-state batteries, advances in understanding have been made by extending the band structure framework to include much more massive Li ions and interstitial vacancies.
Theoretical calculations have studied the role of these charged defects in battery performance, but experimental support for models of interface electronic and chemical changes requires the coupled electronic and chemical measurement of interfaces on a nanometer scale. This has been a challenge due to the interface being deeply embedded in most devices. The most comprehensive experimental studies to date have involved only X-ray photoemission spectroscopy (XPS) valence band emission on a Li/LiPON/LiCO2 (LCO) system.
The physical properties of electrolyte/electrode battery interfaces have been the focus of intense research, particularly within the last decade. In general, there have been considerable advances in understanding and controlling inorganic solid interfaces, including their safety, in the past 20 years. But new techniques are needed to examine the microscopic interphase formation between the cathode and inorganic electrolytes.
The research
The authors introduce a new combination of techniques—X-ray photoemission spectroscopy (XPS) and depth-resolved cathodoluminescence spectroscopy (DRCLS)—to correlate the chemical and electronic structure properties of the electro-chemical cathode/electrolyte interface before and after the ionic transfer process.
XPS is a widely used surface science technique that can measure chemical composition and bonding both at surfaces and depth-resolved using ion beam sputtering. Cathodoluminescence spectroscopy (CLS) and DRCLS, its depth-resolved extension, measure optical emissions among conduction, valence, and defect bands that provide electronic structure information. Together, XPS and DRCLS can correlate chemical composition and electronic structure and their variation with physical location inside solid-state materials on a near-nanometer scale.
DRCLS combined with XPS provides evidence of electronic band structure shifts of V2O5 with the insertion of lithium ions. Without a LiPON coating, these shifts agree with prior predictions and the stoichiometric composition of lithium determined through XPS depth profiling. With the deposition of a LiPON overlayer onto V2O5, the decrease in the split-off DRCLS band indicates that lithium can diffuse from LiPON into the LVO even before electrochemical cycling.
For electrochemically lithiated samples, a pronounced increase in split-off band emission is observed in the LVO near the interface with LiPON, while away from the interface, the split-off intensity is reduced, as expected. Correlated with the DRCLS, XPS-measured Li content is also found to be increased in the LVO near the interface.
Taken together, the authors propose that there is upward band bending in the LVO induced by the interface with LiPON, which may depend on the state of charge. They expect that these interfacial effects are sensitive to the growth and processing methods used to prepare the interface with the potential for altering this behavior with appropriate considerations. For example, specific growth or annealing temperatures or the application of a very thin interfacial layer or treatment may be useful for controlling the interaction between electrons and ions at the interface. DRCLS calibrated with XPS proves to be an effective nondestructive method for probing buried interfaces with nanoscale resolution.
Published May 8, 2023