News Story
Research Review: Combating biofilms with microsystem devices
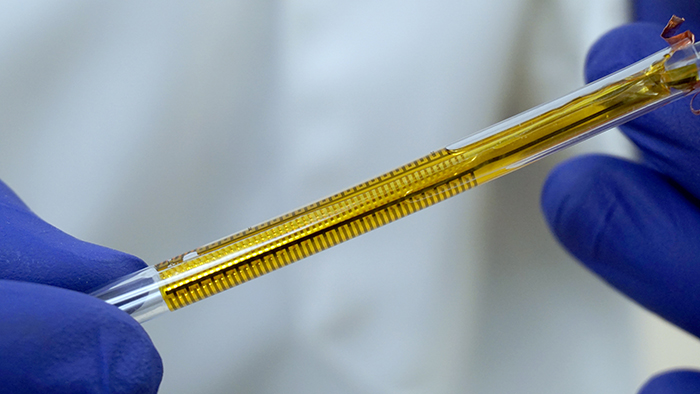
The flexible impedance sensor, shown here inside a clear catheter tube. The sensor's surface (yellow) conforms to the inside of the catheter. The gold comb-like structures are the device's embedded electrodes, which produce the bioelectric effect that works with antibiotics to treat the bacterial biofilm. PHOTO CREDIT: Ryan Huiszoon, MEMS Sensors and Actuators Laboratory, University of Maryland.
A long-term, interdisciplinary research collaboration at the University of Maryland’s A. James Clark School of Engineering has made real progress in creating chemical compounds, developing new kinds of materials, and designing and building microsystems that can both detect and treat these infections.
Bacterial biofilms are almost always involved in device-associated and post-operative infections, and account for approximately half of all the estimated 700,000 to 2 million hospital infections annually. They form when planktonic bacteria adhere to moist surfaces and encase themselves in an extracellular matrix. This matrix offers significant protection for the bacteria against antimicrobial medicines, and also makes it easy for them to spread through gene transfer.
Biofilms easily form on implanted medical devices like catheters, prosthetic joints, and dental implants. Urinary catheters, some of the most commonly inserted medical devices, are susceptible to biofilm forming from bacteria in the urine at a rate of 5–7 percent per day. Because high doses of antibiotics are required for their removal, biofilms contribute to the increase in antibiotic-resistant strains of bacteria.
Doctors need far better ways to detect, measure and effectively treat biofilms.
“There is a need for non-invasive, real-time selective and sensitive detection of biofilms that can aid infectious disease practitioners in the treatment of biofilms on indwelling medical devices,” says Dr. Rodney Donlan of the Biofilm Laboratory at the Centers for Disease Control and Prevention in Atlanta.
In their 10-year collaboration, Professor Reza Ghodssi (Electrical and Computer Engineering; Institute for Systems Research), Professor William Bentley (Fischell Department of Bioengineering), and their students and colleagues have been advancing the state of the art in both biofilm detection and treatment. They have developed in-vitro microsystems that can detect and measure (“characterize”) biofilm, and in-vivo microsystems for biofilm treatment. The most recent device, which can be placed in urinary catheters, can do it all—detect, measure and treat biofilms.
“Our work not only uses microfluidic systems as a discovery platform to investigate biofilm behavior, but also develops future technologies such as flexible substrates and devices that we hope soon will be able to combat the formation or treatment of disease in patients,” Ghodssi says.
New treatment methods for biofilm
With Professors Greg Payne (Fischell Department of Bioengineering), Gary Rubloff (Materials Science and Engineering) and Herman Sintim (Purdue University), the researchers developed treatments that can reduce or eliminate the need for antibiotics.
Chemical compounds called quorum sensing inhibitors can interrupt the pathways by which bacteria communicate, preventing biofilm formation. Bacteria are then maintained in their planktonic state, which keeps them more vulnerable to traditional treatment. “The idea is to shut off communicating cells and make them susceptible to various treatments,” says Bentley.
Electroceuticals use electric fields to generate toxic compounds that kill biofilms and can be used in specialized wound dressings. Surface coatings can include liquid-infused surfaces, micro/nano structured surfaces, or antimicrobial infused surfaces, such as surfaces loaded with silver particles. They are of particular interest in addressing biofilms adhering to devices such as catheters. The bioelectric effect is a small electric field that increases the efficacy of an antimicrobial, such as an antibiotic or quorum sensing inhibitor. Adding it to treatment lowers the amount of antimicrobial needed to be effective, helping slow the increase in antibiotic-resistant bacteria.
The researchers also developed a lab-on-a-chip hydrogel-controlled environment to monitor biofilm activities and test treatments. Hydrogel is a water-based polysaccharide that can help hold bacteria in place. When used within a microfluidic, micro-electro-mechanical (MEMS) platform, the hydrogel permits researchers to assemble bacteria in ways that mimic the formation of a biofilm, then apply stimuli and measure responses in three dimensions.
In 2015, the research team was awarded U.S. Patent 8,952,192 for “Phosphorylated and Branched Dihydroxy-Pentane-Dione (DPD) Analogs as Quorum Sensing Inhibitors in Bacteria.” The patent includes both the compounds and the hydrogel environment.
Milestones in this research
• “A Biofilm Treatment,” 2011[1], explored the potential of coupling DC, AC, and superpositioned potentials and their associated electric fields with antibiotics for biofilm treatment. The energy of the electrical signal—not the type of signal applied—was determined to be the most important element for effective treatment.
• In “AI-2 analogs and antibiotics: a synergistic approach to reduce bacterial biofilms,” 2011[2], Roy, Meyer, Smith, Gamby, Sintim, Ghodssi and Bentley developed new variations of chemical compositions that can inhibit biofilm formation, as well as ways to deliver them to the bacteria. Bacterial cells create an infection by communicating their presence to each other using a signaling molecule called autoinducer 2 (AI-2). At a certain point, the cells sense a “quorum,” which leads to the formation of the biofilm. The new compounds control cells’ quorum-sensing response so that the cells never perceive that they have achieved a quorum and fail to produce the biofilm.
Their synthetic “analogs” of AI-2 can control QS responses, and the researchers tested these in a three-species synthetic ecosystem comprised of the bacteria E. coli, S. typhimurium and V. harveyi. They showed these work among the individual species as well as across the species.
The researchers also developed a “lab-on-a-chip” hydrogel controlled environment in which biofilm activities can be monitored. Hydrogel is a water-based polysaccharide substance that can help to hold bacteria in place. When used within a microfluidic, micro-electro-mechanical platform, the hydrogel permits researchers to assemble bacteria in ways that mimic the formation of a biofilm and apply stimuli and measure responses in three dimensions.
Microsystem devices that can both characterize and treat biofilm
The researchers have developed a number of microsystem approaches to providing sensitive, real-time biofilm measurement. Optical sensing systems measure changes in opacity that accompany biofilm formation in a microfluidic channel. Surface acoustic wave sensors can precisely quantify the mass of a biofilm as it forms on the sensing surface and alters the measured resonant frequency. Microelectrodes quantify biofilm growth in real-time through electrochemical analysis techniques.
Early prototypes of these systems were rigid, planar structures that did not work well with the complex geometries of many types of medical implant devices—for example, the cylindrical shape of catheters—and required bulky components that were not biocompatible. Over the years the researchers have refined the devices to become smaller, biocompatible and flexible.
Milestones in this research
• When a biofilm grows and thickens, it becomes less translucent. In “Development and validation of a microfluidic reactor for biofilm monitoring via optical methods,” 2011[3], a platform was developed that could continuously and non-invasively monitor bacterial signaling and biofilm formation by monitoring the change in biofilm optical density. They built a microfluidic “lab on a chip” containing tiny channels into which fluids can be pumped under highly controlled conditions. On one side of a channel they placed light-emitting diodes (LEDs) and on the other, photodiodes that detect light. They pumped fluid containing E. coli into the channel, and continuously shone light through it. As the biofilm grew in the channel, the photodiodes captured less light. This platform was designed to characterize biofilm formation and response to various drug treatments. Someday, an array of such chips, each programmed for growing infections and testing how they respond to drugs, could be used as a highly controlled, fast and cheap drug development environment.
• The “Micro-BOAT Platform,” 2012[4], demonstrated a microfluidic biofilm observation and treatment device. The platform used an on-chip charge coupled device array that could measure biofilm formation in a microchannel. This was combined with integrated electrodes to apply bioelectric effect-based biofilm treatment. The work demonstrated real-time optical biofilm monitoring and bioelectric effect treatment on-chip. The total biomass reduction by the superpositioned field treatment was 71 percent more than the reduction by only AC or DC treatments. Since the electric field intensity was significantly lower than that required for electrolysis of biological media, this method could be directly applied to in vivo infection treatment.
• Sensors are needed to detect E. coli and other biofilms at very early stages so that physicians can apply drugs more rapidly. Ghodssi and his graduate student Kim built and demonstrated a surface acoustic wave (SAW) sensor for real-time biofilm growth monitoring. The SAW sensor is an extremely thin piezoelectric film made of zinc oxide that can be placed on the surface of a medical device; when a biofilm grows on the film, the sensor’s resonant frequency changes, creating an electrical signal the sensor transmits wirelessly to a physician or patient notification system. The sensor is highly sensitive and biocompatible and its operational frequency meets wireless medical device regulations set by the Federal Communication Commission.
“A bacterial biofilm Surface Acoustic Wave sensor for real time biofilm growth monitoring,” 2013[5], was an integrated system that combined a surface acoustic wave (SAW) sensor for sensitive detection with electrodes for application of the bioelectric effect (BE). This innovation enabled simultaneous biofilm detection, control and treatment, and could be used to monitor and treat biofilms without invasive surgery.
The researchers developed sectioned microfluidic platforms with (2013) and without (2015) valves to test the effectiveness of different biofilm treatments.
• In 2013, Mariana Meyer created a device that used channels with varying depths to accommodate both the biofilms and the tiny pneumatic valves used to separate them. She developed a new technique to create the different levels by alternating layers of photopatterned polymer with thin layers of aluminum oxide within her device mold. The aluminum oxide protects the polymer layer below as a new one is fabricated above. The device was capable of separating the biofilm into isolated sections. This allowed researchers to simultaneously perform multiple tests on the same sample, producing reproducible comparisons of highly variable biofilms. “Biofilm Sectioning Device,” 2013[6].
• “Valveless Bifurcation Sectioning,” 2015[7], used bifurcation microfluidics to generate more reproducible biofilm characterization, by exposing four channels to the same growth conditions in one flow direction and then exposing each of those similar biofilms to a discrete treatment in the opposite flow direction. This device used a single-step fabrication process, increasing simplicity and reliability.
• A 3D-printed characterization platform was developed. This open-channel microfluidic device allows rapid characterization of biofilm growth and response to treatment on 3D-printed polymers. It validates novel anti-biofilm treatments for use with 3D-printed implants and enables further 3D-printed microsystems for biofilm. “3D-Printed Characterization Platform,” 2016[8].
• A sensitive monitoring and feedback-activated bioelectric treatment device was developed. Bifurcation-based microfluidics are combined with an impedance sensor in this device. It enabled sensitive, real-time biofilm monitoring and feedback-activated bioelectric treatment, using the same interdigitated electrodes for both sensing and treatment. “An Integrated Sensor-Treatment System,” 2016[9].
A biofilm sensing and treatment device for catheters
Most recently, the Flexible Impedance Sensor brings many of these advances together in one device. It is a biofilm sensing and treatment microsystem for urinary catheters that combines biofilm sensing and the bioelectric effect treatment in a flexible cylindrical tube. The device is rolled and inserted inside the catheter, where its microfabricated polymeric substrate and electrodes seamlessly conform to the catheter’s surface. The device also has the potential to work on many other kinds of biofilm-susceptible surfaces.
Catheters with this device will be able to simultaneously monitor film formation and implement bioelectric effect-based synergistic treatments to remove them. Its electrodes expose the biofilm to a small electric field which synergistically increases the effectiveness of antimicrobial treatment and significantly lowers the dosage of antibiotics needed for biofilm removal. A patent application has been submitted.
Progress continues
Roger Howe, the William E. Ayer Professor of Engineering in the Department of Electrical Engineering at Stanford University, writes, “In addition to detecting the formation of the biofilm by optical, acoustic, and impedance techniques, this research group has demonstrated that applying electric fields renders the biofilm much more susceptible to antibiotics. In their recent papers, biofilm detection and control have been integrated into the same microsystem platform, which makes the technology easier to use for biofilm control in implantable devices.”
The CDC’s Donlan says, “Drs. Ghodssi, Bentley, and colleagues at the University of Maryland have developed several model systems that can provide sensitive, real-time detection of microbial biofilms, suggesting the potential for new approaches for biofilm surveillance on indwelling medical devices.”
The work is progressing towards multidimensional, challenging environments like detecting and treating biofilm infections in patients or monitoring for biofilm growth in environments like water pipes, according to Ghodssi, with a goal of smart, self-sustaining, flexible sensors.
“We are so excited that these efforts will be among the first devices developed by our new Robert E. Fischell Institute for Biomedical Devices,” says Bentley. “It will bring together these kinds of multidisciplinary approaches and talented individuals to build systems that make a difference.”
Howe concludes, “The impact of this research, as it transitions into biomedical devices, will be to reduce infections while simultaneously reducing the dosage of antibiotics—a major contribution to improving healthcare.”
###
Faculty and students involved
Faculty
Professor Reza Ghodssi (ECE/ISR)
Professor William Bentley (BioE)
Associate Professor Herman O. Sintim (Chemistry & Biochemistry, Purdue University)
Professor Mark Austin (CEE/ISR)
Professor Gregory Payne (Fischell Department of Bioengineering and Institute for Bioscience and Biotechnology Research)
Professor Gary Rubloff (MNE/ISR)
Students and postdoctoral researchers
Varnika Roy (BioE Ph.D. 2011) was advised by William Bentley. She is a scientist at Medimmune.
Jacqueline Smith (Chemistry Ph.D. 2011) was advised by Herman Sintim at the University of Maryland. She is a postdoctoral researcher at Georgetown University.
Matthew Mosteller (MSSE 2012) was advised by Reza Ghodssi and Mark Austin. He is a technology specialist and patent Agent at Fish & Richardson P.C., and a student at the Georgetown University Law School.
Mariana Meyer (BioE Ph.D. 2014) was advised by Reza Ghodss. She is a multifunction engineer in the professional development program at Northrop Grumman Corporation.
Young Wook Kim (EE Ph.D. 2014) was advised by Reza Ghodssi. He is a senior researcher and deputy general manager at Seegene, Inc. in Songpa-gu, Seoul, South Korea.
Hadar Ben-Yoav (Postdoctoral Researcher 2011-2015) is a senior lecturer (assistant professor) at the Ben Gurion University of the Negev in Israel.
Sowmya Subramanian (EE Ph.D. 2016) was advised by Reza Ghodssi. She is now a staff scientist at Choate, Hall & Stewart LLP in Boston.
Ekaterina (Kate) Tolstaya (B.S. EE, B.S. CS 2016) is currently a graduate student at the University of Pennsylvania with an NSF Graduate Research Fellowship. As an undergraduate, she worked on supercapacitors and sensors to monitor biofilm growth.
Ryan Huiszoon (BioE) is a current Ph.D. student advised by Reza Ghodssi.
Funding
National Science Foundation Emerging Frontiers in Research and Innovation (EFRI): Biofunctionalized Devices—On Chip Signaling and "Rewiring" Bacterial Cell-Cell Communication
Robert W. Deutsch Foundation
[1] Young Sook Kim, NPJ Biofilms Microbiomes, 2011
[2] Roy, Meyer, Smith, Gamby, Sintim, Ghodssi and Bentley, Applied Microbiology and Biotechnology, 2012
[3] Meyer, Roy, Bentley and Ghodssi, Journal of Micromechanics and Microengineering, 2011
[4] Kim, Mosteller, Meyer, Ben-Yoav, Bentley and Ghodssi, Hilton Head Workshop, 2012
[5] Kim, Sardari, Illiadis, Wu, Ghodssi, Sensor Actuat A-Phys, 2013
[6] Mariana Meyer, J. Micromech Microeng, 2013
[7] Sowmya Subramanian, Transducers ’15
[8] Ryan Huiszoon, AVS ’16
[9] Sowmya Subramanian, Biomed Microdevices, 2016
Published September 15, 2017